Cylinder accuracy analysis of tangential turning of disk-like workpieces with increased cutting speed
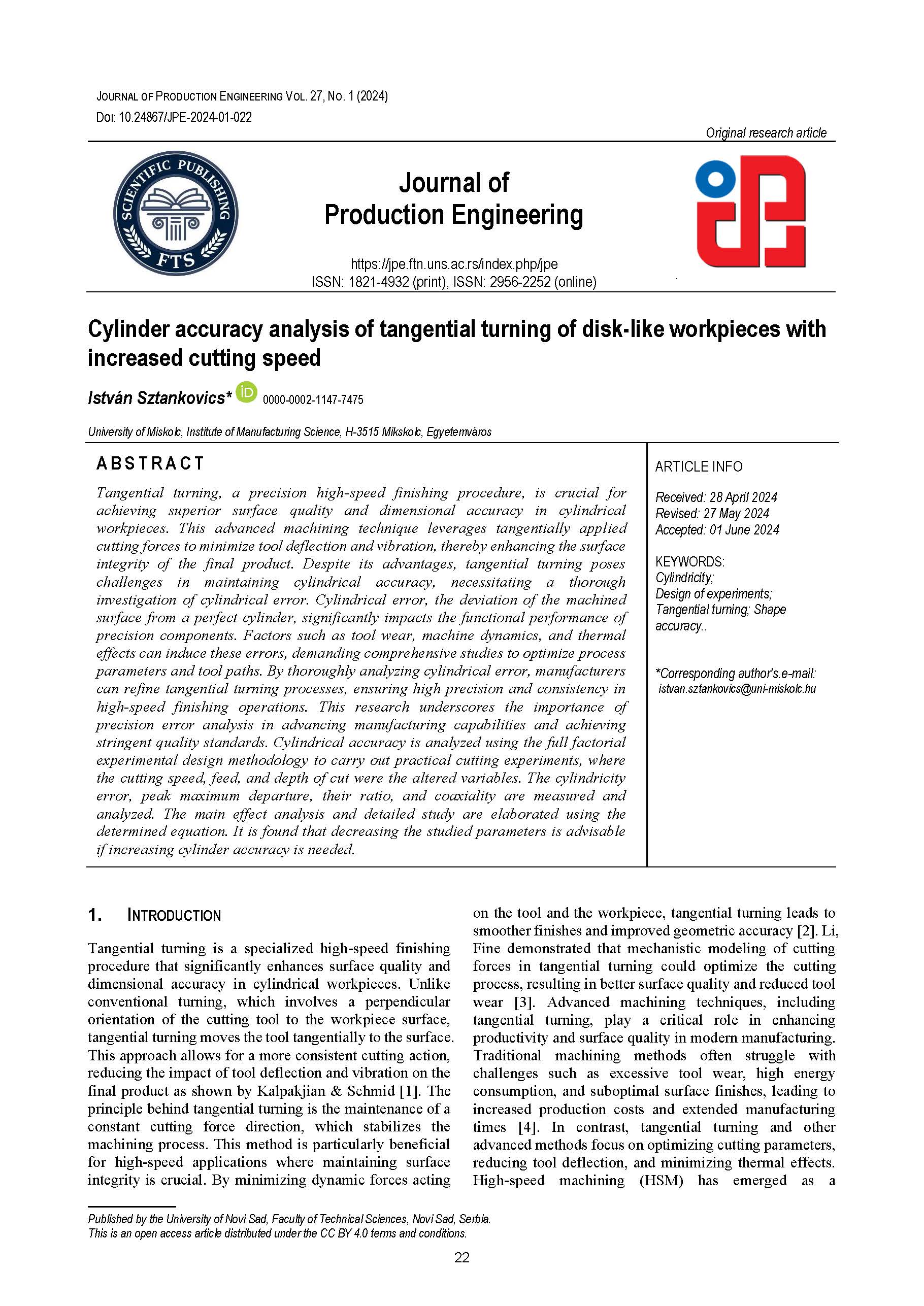
Published 2024-06-23
abstract views: 83 // FULL TEXT ARTICLE (PDF): 0
Keywords
- Cylindricity,
- Design of experiments,
- Tangential turning,
- Shape accuracy
How to Cite
Copyright (c) 2024 Journal of Production Engineering

This work is licensed under a Creative Commons Attribution 4.0 International License.
Abstract
Tangential turning, a precision high-speed finishing procedure, is crucial for achieving superior surface quality and dimensional accuracy in cylindrical workpieces. This advanced machining technique leverages tangentially applied cutting forces to minimize tool deflection and vibration, thereby enhancing the surface integrity of the final product. Despite its advantages, tangential turning poses challenges in maintaining cylindrical accuracy, necessitating a thorough investigation of cylindrical error. Cylindrical error, the deviation of the machined surface from a perfect cylinder, significantly impacts the functional performance of precision components. Factors such as tool wear, machine dynamics, and thermal effects can induce these errors, demanding comprehensive studies to optimize process parameters and tool paths. By thoroughly analyzing cylindrical error, manufacturers can refine tangential turning processes, ensuring high precision and consistency in high-speed finishing operations. This research underscores the importance of precision error analysis in advancing manufacturing capabilities and achieving stringent quality standards. Cylindrical accuracy is analyzed using the full factorial experimental design methodology to carry out practical cutting experiments, where the cutting speed, feed, and depth of cut were the altered variables. The cylindricity error, peak maximum departure, their ratio, and coaxiality are measured and analyzed. The main effect analysis and detailed study are elaborated using the determined equation. It is found that decreasing the studied parameters is advisable if increasing cylinder accuracy is needed.
Dimensions Citation Metrics
References
- Kalpakjian, S., & Schmid, S. R. (2013). Manufacturing Engineering and Technology (7th ed.). Pearson.
- Altintas, Y. (2012). Manufacturing Automation: Metal Cutting Mechanics, Machine Tool Vibrations, and CNC Design. Cambridge University Press.
- Fine, L. (1970). Off centre turning. International Journal of Machine Tool Design and Research, 10(1), pp. 15-24 DOI:10.1016/0020-7357(70)90022-3
- Byrne, G., Dornfeld, D., Inasaki, I., Ketteler, G., König, W., & Teti, R. (1995). Tool condition monitoring (TCM)—the status of research and industrial application. CIRP Annals, 44(2), pp. 541-567. DOI:10.1016/S0007-8506(07)60503-4
- Khare, S. K., & Phull, G. S. (2022). Analysis and optimization of cutting parameters under high-speed machining of Ti-6Al-4V alloy. Materials Today: Proceedings, 62, pp. 4058-4064. DOI:10.1016/j.matpr.2022.04.613
- Schulz, H., Moriwaki, T. (1992). High-speed machining. CIRP Annals, 41(2), pp. 637-643. DOI:10.1016/S0007-8506(07)63250-8
- Davim, J. P. (2001). A note on the determination of optimal cutting conditions for surface finish obtained in turning using design of experiments. Journal of Materials Processing Technology, 116(2-3), pp. 305-308. DOI:10.1016/S0924-0136(01)01063-9
- Schönsleben, P., Vodicka, M., Bunse, K., & Ernst, F. O. (2010). The changing concept of sustainability and economic opportunities for energy-intensive industries. CIRP annals, 59(1), pp. 477-480. DOI:10.1016/j.cirp.2010.03.121
- Sales, W. F., Schoop, J., da Silva, L. R., Machado, Á. R., & Jawahir, I. S. (2020). A review of surface integrity in machining of hardened steels. Journal of Manufacturing Processes, 58, pp. 136-162. DOI:10.1016/j.jmapro.2020.07.040
- El-Hofy, H. (2005). Advanced Machining Processes: Nontraditional and Hybrid Machining Processes. McGraw-Hill Professional.
- Stephenson, D. A., & Agapiou, J. S. (2016). Metal Cutting Theory and Practice (3rd ed.). CRC Press.
- Kundrak, J., Karpuschewski, B., Gyani, K., & Bana, V. (2008). Accuracy of hard turning. Journal of Materials Processing Technology, 202(1-3), pp. 328-338. DOI:10.1016/j.jmatprotec.2007.09.056
- Niaki, F. A., & Mears, L. (2017). A comprehensive study on the effects of tool wear on surface roughness, dimensional integrity and residual stress in turning IN718 hard-to-machine alloy. Journal of Manufacturing Processes, 30, pp. 268-280. DOI:10.1016/j.jmapro.2017.09.016
- Cao, Y., Zhao, X., Li, G., Zong, W., & Sun, T. (2023). Study regarding the influence of process conditions on the surface topography during ultra-precision turning. Journal of Manufacturing Processes, 102, pp. 23-36. DOI:10.1016/j.jmapro.2023.06.066
- Shaw, M. C. (2005). Metal Cutting Principles (2nd ed.). Oxford University Press.
- Venkatesan, D., Kannan, K., & Saravanan, R. (2009). A genetic algorithm-based artificial neural network model for the optimization of machining processes. Neural Computing and Applications, 18, pp. 135-140. DOI: 10.1007/s00521-007-0166-y
- Cheng, K. (2008). Machining Dynamics: Fundamentals, Applications and Practices. Springer.
- Mohamed, A., Hassan, M., M’Saoubi, R., & Attia, H. (2022). Tool condition monitoring for high-performance machining systems—A review. Sensors, 22(6), 2206. DOI:10.3390/s22062206
- Ding, H., Bi, Q., Zhu, L., & Xiong, Y. (2010). Tool path generation and simulation of dynamic cutting process for five-axis NC machining. Chinese Science Bulletin, 55, pp. 3408-3418. DOI:10.1007/s11434-010-3247-7
- Mayr, J., Jedrzejewski, J., Uhlmann, E., Donmez, M. A., Knapp, W., Härtig, F., ... & Wegener, K. (2012). Thermal issues in machine tools. CIRP annals, 61(2), pp. 771-791. DOI:10.1016/j.cirp.2012.05.008
- Wu, G., Li, G., Pan, W., Raja, I., Wang, X., & Ding, S. (2021). A state-of-art review on chatter and geometric errors in thin-wall machining processes. Journal of Manufacturing Processes, 68, pp. 454-480. DOI:10.1016/j.jmapro.2021.05.055
- Esmaeilian, B., Behdad, S., & Wang, B. (2016). The evolution and future of manufacturing: A review. Journal of manufacturing systems, 39, pp. 79-100. DOI:10.1016/j.jmsy.2016.03.001
- Varga, Gy., & Ferencsik, V. (2020). Analysis of cylindricity error of high and low temperature storage tested alternator stators. International Journal of Automotive Technology, 21(6), pp. 1519–1526. DOI:10.1007/s12239-020-0143-3
- Nagy, A., & Varga, Gy. (2021). Effect of abandonment of cooling and lubrication on surface roughness and cylindricity in turning of steel. Multidiszciplináris Tudományok, 11(4), pp. 395–407. DOI:10.35925/j.multi.2021.4.43
- Varga, Gy., Dezső, G., & Szigeti, F. (2022). Shape accuracy Improvement in selective Laser-Melted TI6AL4V cylindrical parts by sliding friction diamond burnishing. Machines, 10(10), 949. DOI:10.3390/machines10100949
- Felhő, Cs., Tesfom, F., & Varga, Gy. (2023). ANOVA analysis and L9 Taguchi design for examination of flat slide burnishing of unalloyed structural carbon steel. Journal of Manufacturing and Materials Processing, 7(4), 136. DOI:10.3390/jmmp7040136
- Adeleke, A. K., Montero, D. J. P., Ani, E. C., Olu-lawal, K. A., & Olajiga, O. K. (2024). Advances in ultraprecision diamond turning: techniques, applications, and future trends. Engineering Science & Technology Journal, 5(3), pp. 740-749. DOI:10.51594/estj.v5i3.869
- Schey, J. A. (2000). Introduction to Manufacturing Processes (3rd ed.). McGraw-Hill Professional.
- Taylor Hobson (2011). Exploring Roundness - A fundamental guide to the measurement of cylindrical form. Taylor Hobson Limited. Leicester, England, pp. 100.
- Trifunović, M., Madić, M., Janković, P. (2023). Analysis of chip compression ratio in turning of polyoxymethylene copolymer. Journal of Production Engineering, vol. 26 (2), pp. 13-17, DOI: 10.24867/JPE-2023-02-013